Power from the Oceans
August 1, 2009
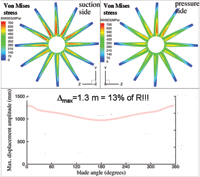
Combining boundary element methods (to model the fluid flow) and finite element methods (to model the structural response of the propeller), Julie Young has simulated the stress and displacement of the blades of a 20-meter turbine. The blades bend by as much as 1.3 meters (13% of their length); such extreme displacements could lead to structural failure. A conventional fluid flow model, treating the propeller blades as rigid rotating bodies, would miss this important design consideration. Figures adapted from Yin Lu (Julie) Young, Department of Naval Architecture and Marine Engineering, University of Michigan, Ann Arbor.
Dana Mackenzie
As long ago as the Middle Ages, people in Ireland, England, and France had the idea of using the ebb and flow of tides to power a water mill. In France, a large dam built in the 1960s at La Rance, in Bretagne, traps water from the tides and is used like an ordinary dam to generate power. In spite of the long history of tidal power, though, this energy source has remained virtually untapped, even as other alternative energy sources have made their way to commercial power grids.
Today, thanks to advances in materials and in mathematical methods for fluid flow analysis, a new generation of tidal energy generators is inching toward deployment---although they often seem to take one step backward for every two steps forward. Meanwhile, devices for extracting energy from surface waves are progressing in a similar advance-and-retreat fashion (see accompanying article). Although neither technology is now commercially viable, supporters believe that both tidal and wave power could follow the same development track as wind power. Within 20 years, they could be generating a significant fraction of the electrical energy produced in the United States, the United Kingdom, and other countries, with an environmental impact far more benign than that of tidal dams.
Tidal Turbine Design
The new breed of tidal energy generators imitate wind turbines, rather than dams. For example, a company called Marine Current Turbines has built a prototype called SeaGen, at Strangford Lough in Ireland---where the first known tidal mill was built in 787 AD! Tidal turbines actually have several advantages over the more familiar wind turbines. First of all, they are not as visible. Moreover, their energy source is steadier and more reliable than the wind--tides, after all, are produced by the highly predictable motion of the moon.
Building a turbine that can survive under water is not as easy as it sounds, however. "The industry thinks that you can just put a beefed-up air turbine in water," says Yin Lu (Julie) Young, an associate professor in the Department of Naval Architecture and Marine Engineering at the University of Michigan, Ann Arbor. But underwater turbines have to cope with several challenges that wind turbines do not. The blades, which move through a medium 850 times denser than air, must be able to withstand much greater forces. Even a moderate increase in flow velocity can add substantially to the load on the blades. They are also subject to corrosion from salt water, and to damage from barnacles and seaweed. Another challenge comes in the design of the supporting structure, which has to hold the turbine in place while contending with both the mobile sea floor and the wind and wave forces at the surface.
Furthermore, underwater turbines are susceptible to "hydrodynamic cavitation," a phenomenon that doesn't affect wind turbines. "I see these turbine designs where the engineers want the blades to spin so fast, and I ask them, don't you have to worry about cavitation?" Young says. "They say, what's cavitation?" (Young, who moves to Michigan from Princeton University in July, presented a paper on marine energy technology, written with Margot Gerritsen, in a SIAM minisymposium on mathematical and computational challenges of global climate and energy processes at the 2009 Joint Math Meetings.)
With experience that includes the design of propellers for naval applications, Young knows all about cavitation. When a propeller blade moves through water fast enough, vapor bubbles will form as the water "boils." (A fluid turns to vapor either when it is heated or when its pressure drops to the vapor pressure of the fluid. It is the latter effect that causes bubbles to form around a propeller, particularly near the blade tip.) Marine engineers have learned to fine-tune the geometry of propeller blades to avoid cavitation, and even to take advantage of it. A specially designed blade can encourage the formation of long, thin, and stable bubbles that extend well beyond the trailing edge of the blade. This phenomenon, called "supercavitation," greatly reduces the water's drag; it has been used in the design of high-speed torpedoes.
For a turbine, though, cavitation is almost always bad. When the bubbles burst, they produce microjets of water that travel at up to 1000 meters per second. "The collapsing of the bubbles damages the blades and generates unwanted noise and vibrations," Young says. "Take a close look at a propeller mounted behind a boat, and you will probably notice a white rim around the edge of the blades, particularly near the tip. That is caused by cavitation erosion." Cavitation also reduces the efficiency of a water turbine, because the turbine is pushing (at least partly) against air rather than against water.
Two Steps Forward, One Step Back
For all these reasons, wind turbines had a huge head start over tidal turbines. But several demonstration projects have been carried out, and more are in the works. SeaGen produced energy at an estimated cost of 16 cents per kilowatt-hour (still far from competitive with fossil-fuel and wind energy), before its blades failed. In 2007, Verdant Power placed six turbines in New York's East River; it was the first multi-turbine array to be successfully connected to the electrical grid and generate power. Verdant also encountered structural problems, however.
Dean Corren, an engineer at Verdant and the creator of the original design, tells the story---a classic example of the "two steps forward, one step back" nature of the alternative-energy biz. "The origins of this turbine go back to the 1980s, with work that I did at New York University," he says. "We built a full-scale prototype, but then alternative energy went to sleep for a long time," as oil prices fell and political support for such research dried up.
"Verdant was founded in 2000, and I joined them in 2003," he recalls. "They had built a copy of my 1985 prototype, with 10-foot fiberglass blades with steel skeletons. We scaled the rotor up to 5 meters for the turbine that was used in the East River. The turbines worked beautifully, but unfortunately, the water velocity was higher than we had measured, and eventually we had rotor failures." Under the unexpectedly high loads, the blades of the rotor folded up.
Verdant tweaked the design, but the new rotor blades also failed. This time, Corren says, "the foundry had done a poor job of casting them, in a subtle way. The shape was done well, but there was too much moisture in the aluminum."
The company is now at work on a fifth-generation turbine. "We are using state-of-the-art modeling of all aspects of the blade casting," Corren says. The new rotors, he says, are working beautifully. In addition, they now use mathematical techniques like the finite element method, which were not used extensively for the first four generations, to model the structures.
Interestingly, Verdant did not have to deal with cavitation in the East River project: The water speed (2.5 meters/second, with peaks of up to 3 meters/second) made it possible to avoid the effect entirely. That may not be the case in other locations, such as the Bay of Fundy or San Francisco Bay, where currents are faster. But those are ideal locations for tidal turbines, because the energy content of a tidal current scales as the cube of the velocity---a current traveling at 4 meters/second will pack four times the power of one traveling at 2.5 meters/second.
State-of-the-Art Math Problem
Designing a rotor that operates efficiently in the presence of cavitation, while maximizing energy capture and avoiding structural failure, is truly a state-of-the-art mathematical problem; in some ways, in fact, it is beyond the state of the art. Young has been working on a flow model that includes all three elements---the water, the blade, and the air bubbles. The motion of the fluid can be modeled as a boundary value problem with boundary conditions of two types, a "no penetration" condition on the solid boundary and a constant-pressure condition on the interface between the water and the bubble. A finite element method is used to solve the structural problem (i.e., the response of the blade). These two problems are fully coupled, allowing the fluid to affect the motion of the blade and vice versa. (Details of the mathematical model, along with research results, can be found in the forthcoming paper "Three-Dimensional Numerical Modeling of the Transient Fluid�Structural Interaction Response of Tidal Turbines," Y.L. Young, M.R. Motley, and R.W. Yeung, Journal of Offshore Mechanics and Arctic Engineering, November 2009.)
To predict the performance of a 20-meter turbine at the Golden Gate Bridge, Young used site-specific tidal velocity data from the Electric Power Research Institute (left) and data from her computer simulations of the performance of the turbine at different velocities. The average current velocity is less than 2 meters per second; because the power output increases as the cube of the current velocity, the peak output occurs during the relatively short intervals when the current velocity exceeds 2 meters per second.
Currently, Young is working on the design of marine turbines with flexible composite materials. Fish swimming through water, with the edges of their fins rippling, offer a good illustration of the advantage of flexibility. "I think there is a lot we can learn from nature, where the structure is not rigid, but able to adapt to the changing environment to achieve optimal performance," Young says. The bending and twisting motion of the turbine blade can be exploited to decrease fatigue and increase the amount of energy captured. This too makes the math more complicated. But it cannot be allowed to get too complicated, Young says---a full transient computational fluid dynamics simulation, coupled with a finite element solver, would take too long to be practical for design work. The trick is to make the right approximations.
Young recently teamed up with Margot Gerritsen and colleagues at Stanford University, who have written software to model the tidal flow in locations like the San Francisco Bay. Combining Gerritsen's models for the choice of the location with her own designs for a better turbine, Young estimates that a 20-meter turbine there could produce 2.7 gigawatt-hours/year---enough to satisfy the electricity demand of more than 200 San Franciscans.
Will this ever happen? It's hard to say. Earlier this decade, the mayor of San Francisco was gung-ho on tidal power, but recently a commission determined that it was not yet cost-effective. For now, the idea is in political limbo.
Elsewhere in the world, however, the political currents are flowing very much in the direction of tidal power. Scotland has announced a �10-million prize for the installation of a commercially viable marine energy technology capable of producing 100 gigawatt-hours over a two-year period. ("They've set the bar so high that we don't see anyone doing that," Corren says.) Scotland has also announced the development of the world's largest tidal energy farm, in Pentland Firth. The reason for Scotland's interest is simple: Pentland Firth has the potential to produce a third of Scotland's total energy requirements. The role for tidal energy in the U.S. is seen as far more modest---the Electric Power Research Institute estimates that tidal energy could provide 3.5% of the total national electricity supply. In coastal regions, however, it could be-come a substantive player.
Both Gerritsen and Young compare the situation of tidal power today to that of wind power in the early 1980s. It took wind energy nearly 30 years to become competitive with fossil fuels in cost and efficiency. Young is optimistic that tidal energy will improve more rapidly than wind did. "It's a baby in terms of technology!" she says.
Dana Mackenzie writes from Santa Cruz, California.